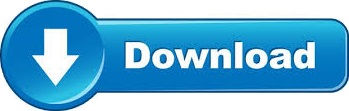
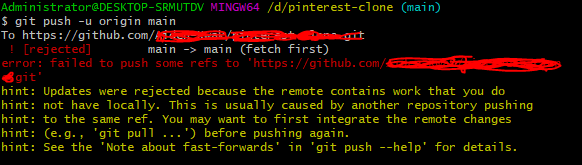
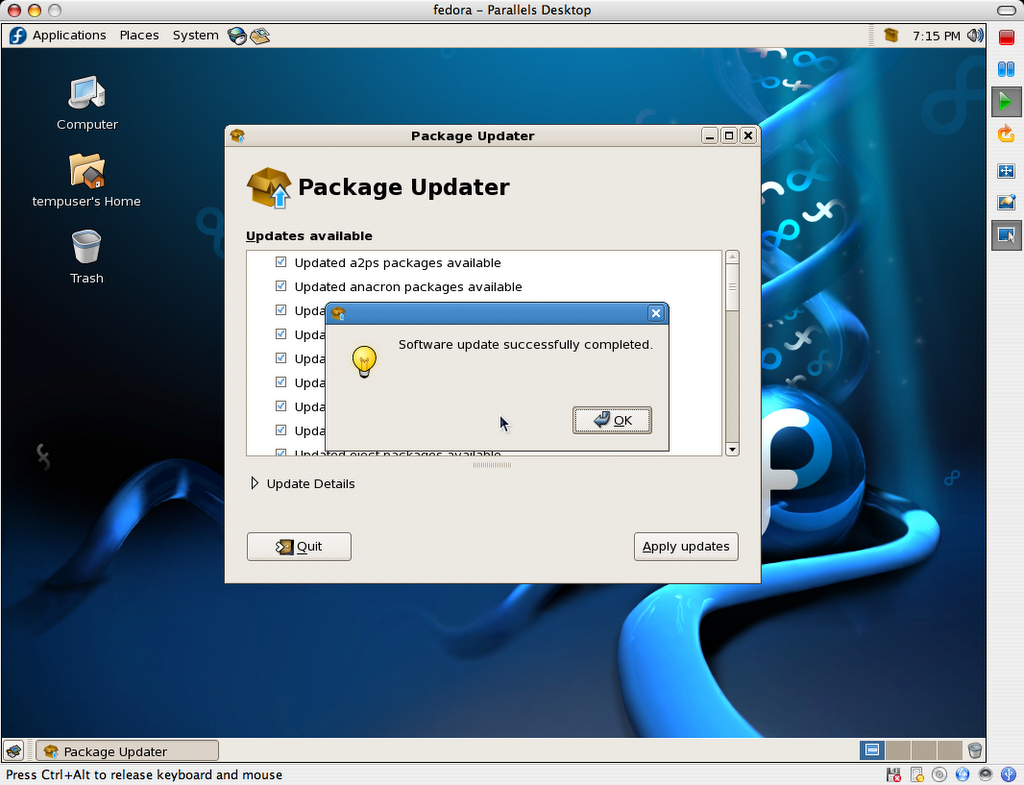
We observe an immediate improvement in electroluminescent external quantum efficiency in our p-i-n LEDs from an average of 9.8% to 13.5%, with a champion efficiency of 15.0%. This has the dual effect of reducing nonradiative recombination at the heterojunction and improving carrier selectivity, which we infer to be due to the inhibition of direct contact between the indium tin oxide and perovskite layers. Here, we show how a thin Al2O3 layer grown by atomic layer deposition can be used to preferentially cover regions of imperfect hole transport layer deposition and form an intermixed composite with the organic transport layer, allowing hole conduction and injection to persist through the organic hole transporter. However, scale-up will be challenging due to the requirement of very thin transport layers for high efficiencies, which often present spatial inhomogeneities from improper wetting and drying during solution processing. Halide perovskite light-emitting diodes (PeLEDs) exhibit great potential for use in next-generation display technologies.
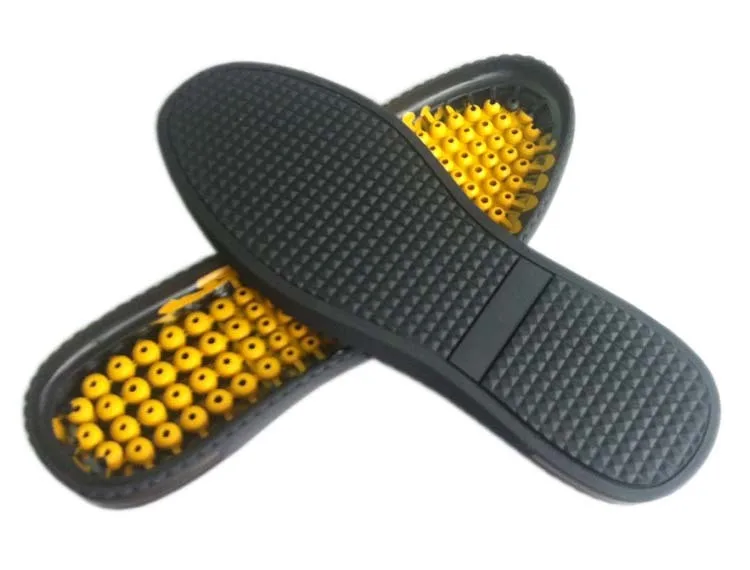
Considering both implementation tactics and application-specific requirements, we identify critical needs to push TTA-UC-based applications from an academic curiosity to a scalable technology.
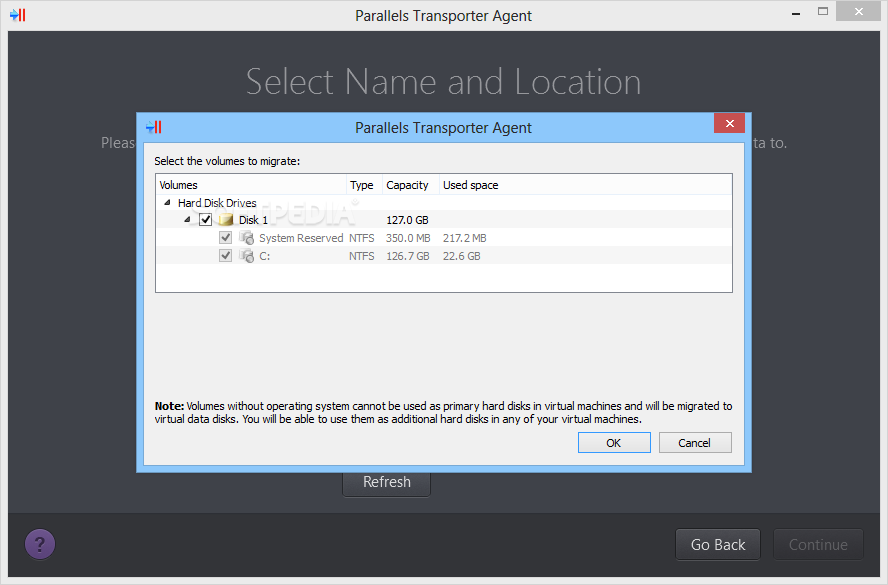
In this Review, we discuss nanoengineering deployment strategies and highlight their uses in recent state-of-the-art examples of TTA-UC integrated in both solution and solid-state applications. With a wide range of sensitizer and annihilator motifs available for TTA-UC, many researchers seek to integrate these materials in solution or solid-state applications. Triplet–triplet annihilation upconversion (TTA-UC), a process which allows for low energy photons to be converted to high energy photons, is poised to overcome these challenges by allowing for precise spatial generation of high energy photons due to its nonlinear nature. Yet delivering photons beyond the surface to many photoresponsive systems has proven challenging, particularly at scale, due to light attenuation via absorption and scattering losses. Using light to control matter has captured the imagination of scientists for generations, as there is an abundance of photons at our disposal. We anticipate this review will be helpful for developing emerging biomaterials for ICB therapy and promoting the clinical application of ICB therapy. Current challenges and future development prospects for expanding the ICB with biomaterials were also summarized. In this review, we reviewed current design strategies for different types of biomaterials to augment ICB therapy effectively and then discussed present representative biomaterial-assisted immune modulation and targeted delivery of checkpoint inhibitors to boost ICB therapy. With the rapid advances in nanotechnology and materials science, various types of biomaterials have been developed to maximize therapeutic efficacy while minimizing side effects by increasing tumor antigenicity, reversing immunosuppressive microenvironment, amplifying antitumor immune response, and reducing extratumoral distribution of checkpoint inhibitors as well as enhancing their retention within target sites. The immune checkpoint blockade (ICB) therapy has revolutionized the field of cancer treatment, while low response rates and systemic toxicity limit its clinical outcomes. We hope that this review can stimulate more insights and inspiration toward the development of electrocatalytic urea synthesis technology. In this review, we present the recent progress on electrochemical urea synthesis based on CO2 and nitrogenous species in aqueous solutions under ambient conditions, providing useful guidance and discussion on the rational design of metal nanocatalyst, the understanding of the C–N coupling reaction mechanism, and existing challenges and prospects for electrochemical urea synthesis. Although an increasing number of studies have made some breakthroughs in electrochemical urea synthesis, the unsatisfactory Faradaic efficiency, low urea yield rate, and ambiguous C–N coupling reaction mechanisms remain the major obstacles to its large-scale applications. The electrochemical coreduction of carbon dioxide (CO2) and nitrogenous species (such as NO3–, NO2–, N2, and NO) for urea synthesis under ambient conditions provides a promising solution to realize carbon/nitrogen neutrality and mitigate environmental pollution.
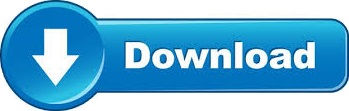